Quantum Properties of Atomic Hydrogen Gas
Atomic hydrogen is the simplest, smallest and lightest of atoms. It is the only element or substance, which remains a gas and does not condense into liquid or solid even at absolute zero of temperature. Furthermore, hydrogen atoms interact with each other on a very short range, when they approach to the distance less than 1 Å.
GRAvity, Spectroscopy and Interferometry with ultra-cold Atoms and Neutrons
In GRASIAN, we are going to re-visit H under extreme conditions of ultralow energies of ~1 peV (~10 nK) never realized before, and attempt to use this system for resolving fundamental questions in particle physics, optical spectroscopy, and QED. First, we are going to trap low-field-seeking (lfs) H magnetically and cool evaporatively to temperatures of ~100 μK following the methods developed by the MIT and Amsterdam groups. Then, we will transfer H in a second trap made from copper conductors. It will improve control of the gas cooling and manipulation of the H cloud at the final stage, when strong trapping fields are not required. Working in the high-density limit at thermodynamic equilibrium, we plan to reach BEC. Studies of properties of the BEC of H is a part of this project. The out-coupled matter-waves from the condensate will be used for interference measurements using the reflection from a superfluid He surface. Then, we propose to explore another region of phase space with low-density non-interacting ensembles of H. Reaching the above-mentioned energy limits is not possible for the gas in thermal equilibrium and high density, because of very slow equilibration rate and large atomic losses in inelastic collisions. Therefore, we will select groups of atoms with lowest vertical or horizontal (or both) velocities. Owing to a very large initial number of H, even after 4-6 orders of magnitude truncation in the velocity space, one may still get the numbers of H, 106-108. The ensemble of ultracold H will be bound by superfluid helium (He) from bottom, gravity from top and small magnetic field gradients in radial directions. We will study GQS of lfs H(D) formed in this trapping geometry. Later, we will use the field of a vertical superconducting wire carrying high current and trapping the high-field-seeking H. Such a trap would provide maximum time of observation of H in GQS. Depending on the success with H and the experience gained, a similar trap can be realized later for anti-H in GBAR project in CERN, also for n, which require ~103 times higher magnetic fields. Several options for precision optical and HF spectroscopy exists using ensembles of H falling down in the field of gravity. These tasks could be solved only due to SYNERGY between the members of the GRASIAN team.
In the GRASIAN collaboration our group is responsible for creating a source of H atoms with ultra-low velocities. We will trap and cool a large ensemble of atoms using well known methods of magnetic trapping and evaporative cooling. During 2019 and 2020 we have been building a large Ioffe-Pritchard trap with the inner diameter of 11 cm and length of 40 cm. Radial compresion is provided by 8 race-track coils in the octupole configuration. The magnets of the trap were tested in summer 2020, and at 4.2 K reached the barrier height of 0.85 T. Then, in spring 2021 we installed the trap into a cryostat with a dilution refrigerator, where operates in vacuum at 1.5 K. Tests of the trap at these conditions demonstrated nearly same behavior as in the bath of liquid 4He. Descriptiopn of our Ioffe-Pritchard trap and report on the test results are available in the recent preprint (arXiv:2108.09123, accepted for publication in the Review of Scientific Instruments). We plan to trap and cool >10<sup>14</sup> of H atoms to temperatures below 1 mK.
After that we will transfer the H cloud into the second smaller trap with more accurate and fast control of the trapping field. We plan to reach quantum degeneracy and BEC in the second trap (T2). Working with the H gas out of thermodynamic equillibrium we will select the atoms with smallest (vertical) velocities and deliver them to numerous fundamental experiments planned by the members of the GRASIAN team. These will include study of the quantum behavior in the field of gravity near the surface of superfluid heium, Quantum Reflection (QR) and observation of the Gravitational Quantum States (GQS), precise 1S-2S optical spectroscopy and Ramsey Interferometry, accurate measurement of hyperfine transition frequencies.
Coils of the large (T1) and second smaller trap (T2) are illustrated in the figure below. T2 has variable configuration between quadrupole, sextupole and 12 pole and the trapping field order of 20 Gauss. Thanks to large available space, we choose a different coil geometry to avoid alternating off-axis fields at the trap ends.
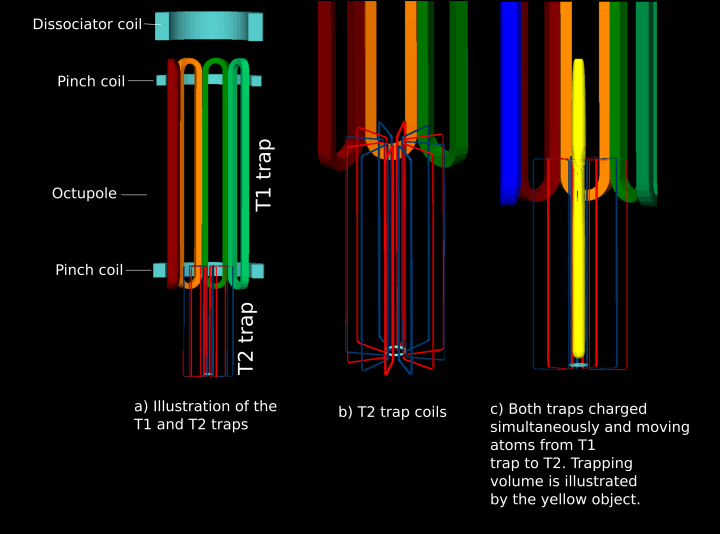
At the same time with the development and tests of the magnetic trap we build a UV laser system for diagnostics of the trapped gas and precision spectroscopy of ultra-low energy H. We will use two-photon spectroscopy of the 1S-2S transition at 243 nm. Optical system required for these experiments is under construction together with our colleagures N. Kolachevsky and A. Golovizin from Lebedev Insitute (Moscow). It will be based on cavity stabilized diode laser followed by two frequency doubling stages. We are aiming on reaching 50 mW of CW radiation at 243 nm with the stability better than 10Hz. We hope that our laser source will allow experiments approaching the limit of the 1S-2S natural linewidth 1.3 Hz. We plan to complete laser system in spring 2022 and get first spectra of the trapped H gas in summer 2022.